![]() | ![]() | ![]() | ![]() | ![]() |
---|---|---|---|---|
![]() | ![]() | ![]() | ![]() | ![]() |
![]() | ![]() | ![]() | ![]() | ![]() |
![]() | ![]() | ![]() | ![]() | ![]() |
![]() | ![]() | ![]() | ![]() | ![]() |
![]() | ![]() | ![]() | ![]() | ![]() |
The future of Arctic sea-ice biogeochemistry and ice-associated ecosystems
The Arctic sea-ice-scape is rapidly transforming. As a consequence of climate changes, sea ice is expected to generally become thinner, younger and more ephemeral than before. This Perspective assesses potential changes for key sea-ice climatic, biogeochemical and biological properties as well as processes in response to environmental changes.

Sea ice biogeochemical processes and associated ecosystem (Lannuzel et al., 2020)
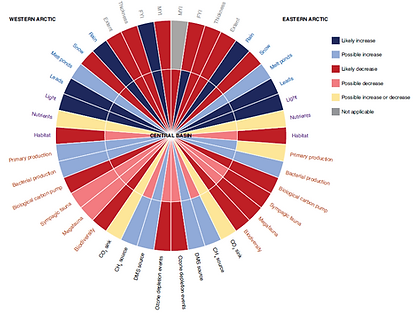
Future of sea ice biogeochemical processes and associated ecosystem (Lannuzel et al., 2020)
Sea Ice nutrient distributions in East Antarctica
Iron (Fe) plays a crucial role in microalgal physiology and can control their growth in the Southern Ocean, where Fe concentrations are naturally low. Antarctic sea ice can incorporate high levels of Fe during its formation triggering phytoplankton blooms at the sea-ice edge during the melt season. No studies to date have assessed sea-ice Fe distributions in East Antarctica during mid- to late summer.
In this study, we discuss Fe distribution in parallel with key sea-ice physical and biological parameters measured during an expedition to East Antarctica in summer 2016/2017 to answer our central question: is Fe limiting sea-ice primary productivity during summer?
Our results suggest nitrate, rather than Fe is the key nutrient controlling sea-ice algal growth at this time of the year. We also found Fe-rich platelet ice incorporated underneath pack ice sampled near the Moscow University Ice Shelf which suggests the potential accretion of Fe-rich ice shelf waters under the sea ice.
As climate change is expected to accelerate Antarctic ice shelve melting, a better understanding of how increased rates of glacial meltwater discharge will impact the distribution of Fe within the sea ice during summer is needed.

Sea ice nutrients in East Antarctica (Duprat et al., 2020)
Sea Ice CO2 Dynamics Across Seasons in McMurdo, Antarctica
Sea ice participates actively in the regional cycling of CO2 both as a source and a sink at different times of the year depending on ice physics, ice chemistry, and ice trophic status (autotrophic vs. heterotrophic). We identified the key processes driving the CO2 dynamics in each sea ice layer (surface, interior, and bottom) from McMurdo Sound (Antarctica) from late winter to summer.
At the surface, CO2 release from the ice to the atmosphere occurred in late winter while CO2 uptake occurred in summer. Superimposed upon this seasonal pattern, we observed a diurnal pattern with both release and uptake occurring over 24 hr period. This diurnal pattern can be related to physical processes (nocturnal freeze‐up and diurnal melting) or biotic processes (autotrophy or heterotrophy).
In the ice interior, a succession of autotrophic and heterotrophic phases took place.
At the sea ice bottom, a particular assemblage of microbial cells and organic matter, called biofilm, enabled the accumulation of biomass and nitrate plus nitrite simultaneously leading to both autotrophic and heterotrophic activities.
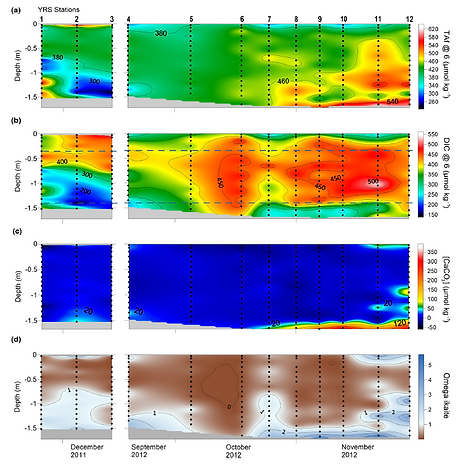
Sea ice Carbonates at YROSIAE (van der Linden et al., 2020)
Winter sea ice biogeochemistry under warming and more snow
In this study, we present the results of physical and biogeochemical investigations on winter Antarctic pack ice in the Weddell Sea (R. V. Polarstern AWECS cruise, June–August 2013) which are compared with those from two similar studies conducted in the area in 1986 and 1992.
The winter 2013 was characterized by a warm sea ice cover due to the combined effects of deep snow and frequent warm cyclones events penetrating southward from the open Southern Ocean. These conditions were favorable to high ice permeability, favoring relatively high chlorophyll-a (Chl-a) concentrations.
Large-scale sea ice model simulations also suggest a context of increasingly deep snow, warm ice, and large brine fractions across the three observational years, despite the fact that the model is forced with a snowfall climatology.
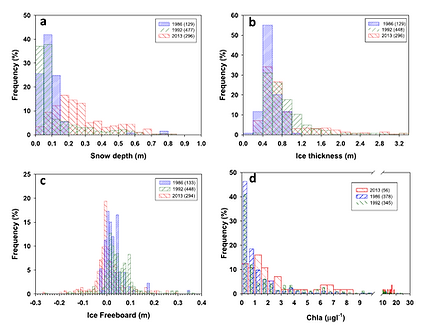
Observed sea ice (Tison et al., 2017)
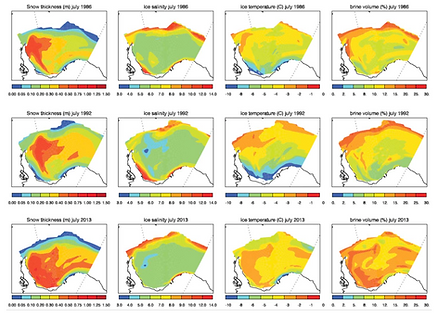
Modelled sea ice LIM3 (1986, 1992, 2013; Tison et al., 2017)
Sea ice carbon pump
For the first time, we assessed the role of sea ice physics and biogeochemistry in pumping atmospheric CO2 with a Global Earth System Model. To do so, we added a first-order representation of DIC and TA storage and release in/from sea ice.
Our results suggest that sea ice releases several hundreds of Tg C per year, but only a small fraction (2%) is exported to depth in the Arctic and Southern Oceans Bassins.
Sea ice biogeochemistry (CaCO3 precipitation, ice-air CO2 fluxes and sea ice NPP) modifies ocean-atmosphere CO2 fluxes by a few Tg C yr-1 in the sea ice zone. The Arctic becomes a smaller pre-industrial CO2 sink and the Southern Ocean a smaller pre-industrial CO2 source.
The representation of these processes could differ substantially if a higher resolution or different ocean model were used.
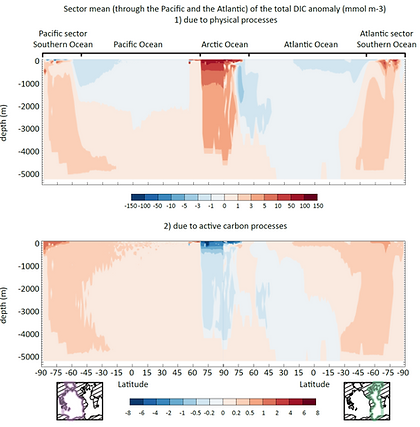
Sea ice CO2 model (Moreau et al., 2016)
Sea ice carbon dynamics
We designed a sea ice halo-thermodynamics model that resolves CO2 dynamics, including ice-atmosphere CO2 fluxes, ikaite precipitation and primary production and respiration.
The model shows that DIC dynamics in sea ice are controlled by physical processes at the first order and biogeochemical processes at second order.
CO2 fluxes as given by the model agree with in situ observations obtained with bell-chambers, but disagree with observations obtained from eddy-covariance. The results suggest that eddy-covariance fluxes may represent other processes or sources of CO2, such as wind-pumping of CO2 in snow, surface communities, or CO2 fluxes from leads or cracks.
Ikaite precipitation in the model is limited to ~400 mmol m-3 by TA stocks in sea ice. High simulated TA/DIC ratio (~2) are consistent with observations but suggest outgassing rather than ikaite precipiation.
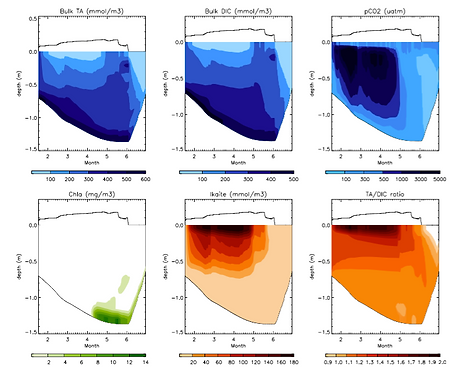
Sea ice CO2 model (Moreau et al., 2015)
Sea ice gas dynamics
Before that, we included gas physics in our sea ice model (LIM1D).
In the model, gas bubbles nucleate within sea ice when argon (Ar) is above its saturation and when the total gas pressure inside brines (including N2, O2 and Ar) is above the brine hydrostatic pressure.
Gs bubbles rise buoyantly to the surface of the ice when the brine network is connected. This implies that brine volume is above a given threshold of 10%.
Ice-atmosphere Ar fluxes are formulated as a diffusive process proportional to the differential partial pressure of Ar between brine inclusions and the atmosphere.
This study showed that gas bubbles may indeed account for >50% of the total gas content inside sea ice. When sea ice warms, gas bubbles could also be responsible for a large pulse flux to the atmosphere.

Sea ice gas model showing the importance of gas bubbles (Moreau et al., 2014)
Assessing O2 dynamics under sea ice
Given that gas physics and carbon dynamics are now represented, the model can be used to look at specific processes. During the INTERICE 5 experiment, sea ice was grown without primary producers in an indoor facility in Hambourg (Germanny). Besides gas physics, bacterial respiration was the only biological process that influenced O2 dynamics in/under sea ice.
To adequately represent the evolution of O2 under sea ice with the model, bacterial respiration was required. In addition, with this analysis, we calibrated bacterial growth efficiency (BGE) under sea ice to be between 0.4 and 0.5, suggesting a rather important role of bacterial respiration in polar ecosystems.

Obeserved and modelled O2 under sea ice during the INTERICE 5 experiment (Moreau et al., 2016)
Drivers of ice-air CO2 fluxes
We measured ice-air CO2 fluxes during the ice growth experiment INTERICE 5 and reproduced them with the model.
Cooling seawater prior to sea ice formation acted as a sink for atmospheric CO2. During the ice growth phase, sea ice turned to a source of CO2. During ice decay, sea ice shifted back again to a sink of CO2.
One of the striking feature was the inability of the model to represent the amplitude of ice-air CO2 fluxes during the ice growth phase, if using only the dissolved gas pathway.
This limitation by the model is due to the slow transport of dissolved inorganic carbon through cold sea ice. The best means we found to explain the high air-ice carbon fluxes during ice growth is an intense yet uncertain gas bubble efflux, suggesting an important role of gas bubbles in ice-air gas fluxes.

Obeserved and modelled ice-air CO2 fluxes during the INTERICE 5 experiment (Kotovitch et al., 2016)
Degradation of rivers' organic matter increases pCO2 in Arctic sea ice
We grew sea ice from both seawater (SW) and seawater + river water (SW+RW) enriched in dissolved organic matter. This experiment was done to understand the role of excess organic matter on sea ice biogeochemistry. We hypothesized that the higher pCO2 measured in Arctic sea ice compared to Antarctic sea ice is due to the degradation of organic matter by bacteria.
Using both our observations and modelling experiment, we could confirm that the presence of excess organic matter in seawater prior to freezing led to higher pCO2 in sea ice. Bacterial respiration was the main driver for this accumulation of CO2 in sea ice, while air-ice and ice-seawater CO2 fluxes acted oppositely.
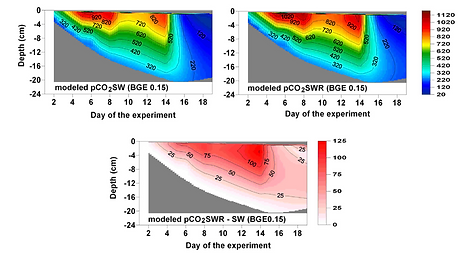
Modelled sea ice pCO2 for the SW and SW+RW treatments during the INTERICE 5 experiment (Zhou et al., 2016)